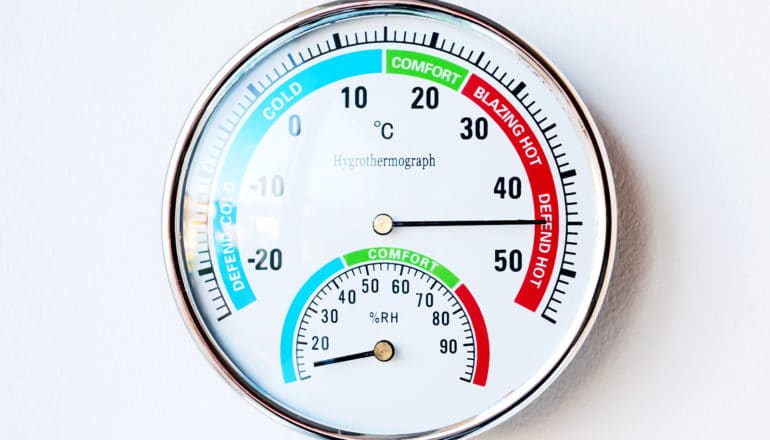
The first measurement of heat transfer through a single molecule may be a step towards molecular computing.
Molecular computing involves building circuits up from molecules rather than carving them out of silicon as a way to max out Moore’s Law and make the most powerful conventional computers possible.
Moore’s Law began as an observation that the number of transistors in an integrated circuit doubles every two years, doubling the density of processing power. Molecular computing is widely believed to be Moore’s Law’s end game, but many obstacles stand in the way, one of which is heat transfer.
Molecular computing’s heat problem
“Heat is a problem in molecular computing because the electronic components are essentially strings of atoms bridging two electrodes. As the molecule gets hot, the atoms vibrate very rapidly, and the string can break,” says Edgar Meyhofer, professor of mechanical engineering at the University of Michigan.
Until now, the transfer of heat along these molecules couldn’t be measured, let alone controlled. But Meyhofer and Pramod Reddy, also a professor of mechanical engineering, have led the first experiment observing the rate at which heat flows through a molecular chain.
“While electronic aspects of molecular computing have been studied for the past 15 or 20 years, heat flows have been impossible to study experimentally,” Reddy says. “The faster heat can dissipate from molecular junctions, the more reliable future molecular computing devices could be.”
The experiment
Meyhofer and Reddy have been building the capability to do this experiment for nearly a decade. They’ve developed a heat-measuring device, or calorimeter, that is almost totally isolated from the rest of the room, enabling it to have excellent thermal sensitivity. They heated the calorimeter to about 20 to 40 Celsius degrees above the room temperature (68–104 degrees F).
The researchers equipped the calorimeter with a gold electrode with a nanometer-sized tip, roughly a thousandth the thickness of a human hair. The team and another team from Kookmin University, visiting Ann Arbor from Seoul, South Korea, prepared a room temperature gold electrode with a coating of molecules (chains of carbon atoms).
They brought the two electrodes together until they just touched, which enabled some chains of carbon atoms to attach to the calorimeter’s electrode. With the electrodes in contact, heat flowed freely from the calorimeter, as did an electrical current. The researchers then slowly drew the electrodes apart, so that only the chains of carbon atoms connected them.
Over the course of the separation, these chains continued to rip or drop away, one after the other. The team used the amount of electrical current flowing across the electrodes to deduce how many molecules remained. Collaborators from Japan and Germany had calculated the current expected when just one molecule remained—as well as the expected heat transfer across that molecule.
Capturing heat flow
When a single molecule remained between the electrodes, the team held the electrodes at that separation until it broke away on its own. This caused a sudden, minuscule rise in the temperature of the calorimeter, and from that temperature increase, the team figured out how much heat had been flowing through the single-molecule carbon chain.
They conducted heat flow experiments with carbon chains between two and 10 atoms long, but the length of the chain did not seem to affect the rate at which heat moved through it. The heat transfer rate was about 20 picowatts (20 trillionths of a watt) per degree Celsius (1.8 degree F) of difference between the calorimeter and the electrode held at room temperature.
“In the macroscopic world, for a material like copper or wood, the thermal conductance falls as the length of the material increases. The electrical conductance of metals also follows a similar rule,” says first author Longji Cui, a PhD graduate who is currently a postdoctoral researcher in physics at Rice University.
“However, things are very different at the nanoscale,” Cui says. “One extreme case is molecular junctions, in which quantum effects dominate their transport properties. We found that the electrical conductance falls exponentially as the length increases, whereas the thermal conductance is more or less the same.”
Theoretical predictions suggest that heat’s ease of movement at the nanoscale holds up even as the molecular chains get much longer, 100 nanometers in length or more—roughly 100 times the length of the 10-atom chain tested in this study. The team is now exploring how to investigate whether that is true.
This study appears in the journal Nature. Additional researchers from the University of Konstanz in Germany and the Okinawa Institute of Science and Technology Graduate University in Japan contributed to the work.
Funding for the study came from the US Office of Naval Research, the Department of Energy, the National Science Foundation, the Korean National Research Foundation, and German Research Foundation.
Source: University of Michigan
The post Hot experiment brings molecular computing one step closer appeared first on Futurity.
from Futurity https://ift.tt/2YjKLZ1
No comments:
Post a Comment